Difference between revisions of "Adaptive Behavior and Learning/contents/Chapter 1"
m (removed "editing" notice) |
m (put main contents in a section, so "edit notes" would be outdented properly) |
||
Line 3: | Line 3: | ||
[http://psychweb.psych.duke.edu/department/jers/abl/Chapter01.pdf PDF] | [http://psychweb.psych.duke.edu/department/jers/abl/Chapter01.pdf PDF] | ||
+ | =Contents= | ||
==NICHES: SIMILARITIES AND DIFFERENCES== | ==NICHES: SIMILARITIES AND DIFFERENCES== | ||
Organisms are machines designed by their evolution to play a certain role. This role, together | Organisms are machines designed by their evolution to play a certain role. This role, together |
Revision as of 20:11, 11 August 2005
Adaptive Behavior and Learning: Chapter 1
Contents
NICHES: SIMILARITIES AND DIFFERENCES
Organisms are machines designed by their evolution to play a certain role. This role, together with the environment within which it is played, is called the organism’s niche1. For example, most cats  tigers, leopards, mountain lions  play the role of solitary hunters; wolves and wild dogs are social hunters; antelope are social grazers; and so on. The niche defines the patterns of adaptive behavior essential to an animal’s survival and reproduction.
For simple niches, such as those filled by most nonsocial invertebrates, direct responses to particular kinds of stimulation are all that is required. The animal need keep no record of its past history in order to succeed; it need only avoid bad things and approach good ones. A modest memory for the immediate past allows the creature to respond to changes in stimulation. Direct stimulus-response mechanisms, plus some sensitivity to rates of change, are sufficient for a wide range of surprisingly intelligent behavior. Adaptive mechanisms that require little or no dependence on history are discussed in Chapters 2 and 3.
As the niche grows more complex, adaptive behavior depends more and more on the animal’s past. The greater flexibility that this allows carries with it two kinds of cost: First, the animal must have a past if its behavior is to be guided by it. This implies a lengthening of infancy and adolescence, which necessarily delays reproductive maturity, and puts the individual at a reproductive-fitness disadvantage compared to others quicker on the draw – it is sometimes better to be dumb and fast than intelligent and slow. Second, there is a growing bookkeeping cost. The behaviors acquired through past experience, and some representation of the environ- ments in which they are appropriate, must be “stored,†with minimal duplication, in such a way that the animal has ready access to the most appropriate action. Representing data in the most flexible and economical way is a problem that also confronts human filing systems. Much work in computer science is concerned with “data-base management,†as this is termed. The difficulties encountered in designing efficient and flexible data-base-management systems show that early learning theories greatly underestimated the information processing task implied by the behavior of mammals and birds.
Situations rarely recur in precisely the same form; and only some of the differences between situations are important for action. Hence, the animal’s representation of past environments must also allow it to behave appropriately in environments similar to those it has already encountered. Just what similar means, and how it is determined both by the animal’s evolutionary history and its own experience, is one of the most intriguing unsolved questions in animal behavior. These issues are taken up in Chapters 10, 13, and 14.
When niches grow more complex, the need for simple mechanisms does not diminish – even human beings need reflexes, for example  but, in addition, more complex, history-dependent processes are required.
An animal’s past experience can affect its future in a variety of ways. The simplest way to make sense of these is the supposed dichotomy between learned and innate behavior. Innate behavior is completely independent of experience, and learned behavior is, well, learned. Of course, nothing is truly innate, in the sense of being independent of any experience, but many things are almost independent of any particular kind of experience. For example, many small invertebrates avoid light; they need no special training, no nasty shock in a lighted place, to show this pattern. Most mammalian reflexes are of this sort: As soon as an infant can move at all, it will automatically withdraw its hand from the fire. The knee jerk to a tap, pupillary contraction to a bright light, and many other reflexes are all concomitants of development in a variety of environments, common to all normal members of the human species. I discuss reflexes in Chapter 2.
But there are many effects of experience that do not fit into the innate-learned dichotomy. For example, age slows responses and hardens joints, fatigue reduces muscular strength, hunger (food deprivation) and thirst change preferences in systematic, reversible ways, and so on; a number of other, developmental effects will be discussed shortly. None of these corresponds to the usual meaning of the term learning, which refers to a more specific and only partly reversible change, often related to a positive or negative outcome: The animal learns where food is to be found or to avoid the predator. This book is primarily concerned with learning in this sense, but the category is not exact  simply because we do not really know what learning is. Indeed, there is probably no single process that underlies it. Experience can change behavior in many ways that manifestly do not involve learning, as well as in ways where we are not sure. In other words, there is no hard-and-fast line separating learning from other kinds of behavioral change. There is no neat dichotomy between “learned versus innate†behavior; rather, there is a spectrum of ways in which past experience affects future behavior, and learning is perhaps the most interesting, and certainly the least understood, of these.
The innate-learned dichotomy, nevertheless, refers to a useful distinction better expressed by the term canalization. A structure or behavior is said to be canalized if its development is almost independent of a particular experience or environment. Some things develop in almost any environment: Characteristics such as the four-chambered heart of mammals, or bilateral symmetry, are strongly canalized, in the sense that just about any environment that allows the organism to develop at all will also be sufficient to permit them. A trait such as competence in the English language, or the ability to do algebra, is not canalized at all, because it is critically dependent on a particular environment. Competence in some language is an intermediate case: Evidently just about any linguistic environment is sufficient to produce language learning in a normal infant, even in the absence of explicit instruction. Striking proof of this was offered recently by a school for the deaf in Nicaragua1. Taught in school only to lip read, the pupils nevertheless developed entirely on their own a highly structured sign language. In a similar way, male chaffinches and white-crowned sparrows will develop some adult song if they can listen to a model at the critical time in their first year of life, but the kind of song they develop depends on the model, as well as the species. Language and song development are canalized, but the particular song or language to be learned is not.
What an animal learns, and the way that it learns it, is much affected by its niche. Because niches differ in many respects, so, too, do learning mechanisms. Since niches do not differ in every respect, there are also similarities among learning mechanisms.
Space and time are common to all niches. In consequence, a wide range of animal species adapt to the temporal and spatial properties of the environment in similar ways. There are some general rules that apply across niches: Old information is generally less useful than new information; consequently animals forget, and they forget less about things they have learned recently. Conversely, the environment of an animal around the time of birth usually has a special significance, and things learned at that time may be especially resistant to change. Food, water, sex, and habitat are vitally important to all species. Hence these things are better remembered than “neutral†events and have special properties as guides of behavior.
This book is mainly concerned with the way that animals adapt to these things that are common to all niches2. The major emphasis is on adaptation that depends on learning about rewards and punishments.
PHILOSOPHICAL BACKGROUND
Methodological behaviorism
Animals and people seem to have purposes, beliefs, attitudes, and desires; they seem to know some things and not others, to want some things and disdain others, and so on. These are what philosophers call intentional systems. Intentionality may seem to set psychology apart from the physical and biological sciences. After all, the chemist does not worry about the beliefs of his compounds nor is the physicist concerned about the purposes of protons or the quirks of quarks. Does this mean that psychology is not scientific? Does it mean that it is different in kind from the physical sciences? Not at all; the difference is in the richness of behavior of the things studied, their sensitivity to their environment, and the dependence of present behavior on past experience. The language of intentionality is simply the everyday way that we deal with complex historical systems. I typed the first edition of this book with the aid of a microcomputer that had an ancient operating system called “CP/M.†Look how the instruction manual refers to CP/M and its associated programs: “CP/M could not find a disk…†“PIP assumes that a... character†“CP/M does not know that...†“Seven commands are recognized by CP/M.†No one assumes that there is a little man or woman, complete with “real†knowledge, beliefs, desires, and understanding, inhabiting the microchips. Anything that responds to varied stimuli in varied ways, especially if its behavior depends upon past history, is understood at a commonsense level in intentional terms.
The most striking examples are provided by chess-playing programs. A good one elicits precisely the same kinds of comment we would use for a human player: “It is attacking the queen,†“It’s trying to get control of the center of the board,†and so on. Yet no one doubts that the underlying program provides a perfectly precise and mechanical account of the machine’s behavior3.
But do machines really have beliefs, attitudes, and so on? Aren’t we just begging the question by talking about smart machines? There are two questions here: First, are there such “things†as beliefs, desires, and so forth? And second, if so, do machines possess them? There are two schools of thought on these questions: the first answers “yes†to the first question and “no,†or at least “probably not,†to the second; the second views the questions as irrelevant. Human beings have real desires, attitudes, and so on, the first view holds, and it is the business of real psychologists to study them. Sometimes attitudes and beliefs are deemed to be worthy of study in their own right. More commonly, perhaps, they are studied as causes of action; people do what they do because they believe what they believe. This approach leaves little room for work with nonhuman animals, tends to keep things at a verbal level, and attends first to the “meaning†of people’s actions, verbal and otherwise, rather than focusing on the details of the actions themselves. This is the psychology of paper-and-pencil test, of interview, and of verbal report.
The practical utility of this view cannot be denied. The power of advertising rests, in some measure, on the correct assessment of people’s attitudes to, for example, bodily functions and products that promise to diminish, enhance, or in some other way modify them. Nevertheless, it has both experimental and theoretical limitations. The experimental problem derives from the difficulty of separating correlation from causation. This is an old question: Do we run because we are afraid, or are we afraid because we run? “Fear†is a property of the subject’s internal state, not something external that the experimenter can manipulate directly. Consequently, one can never be certain that the running and the fear are not both caused simultaneously by the same external conditions. The problem is not insuperable. There are ways that intentional terms like “fear,†“hope,†and so on can be made methodologically respectable and tied to observables. The theoretical question is whether the labor involved is worth it. The whole enterprise rests on the presupposition that familiar intentional terms such as “fear,†“belief,†“attitude,†and the like form the very best basis for theoretical psychology. Yet our discussion of clever computer programs showed that such terms represent a primitive kind of explanation at best. They enable someone ignorant of the details of the program to make some sort of sense of what the machine is doing. But full understanding rarely reveals anything in the program that corresponds directly to intentional terms, useful though they may be in the absence of anything better. It is rash, therefore, to base a program of psychological research on the assumption that intentional terms represent the ultimate form of explanation.
Thus, the second answer to the question “Are there really such things as beliefs, desires and so on?†is “Maybe... but who cares?†Obviously these terms are useful ways of coping with some complex systems. But the “really†question is metaphysical, and we have no reason to suppose that these terms will prove especially useful in unraveling the mechanisms of behavior  which are what we are really interested in. Hence, we will be concerned with the behaviors of people and animals, measured pretty much in physical terms  that is, with a minimum of interpretation. This is termed methodological behaviorism, and is the dominant stance among psychologists and biologists interested in animal behavior4.
Methodological behaviorism is not untheoretical; it simply takes no advance position on the nature of appropriate theory. In particular, it does not presume that psychological theory should be based on intentional language.
Two kinds of explanation
The ultimate explanation of the chess-playing program is in terms of the program itself, the individual instructions that determine each move as a function of prior moves by both players. But it is usually convenient, when designing such a program as well as when trying to understand it, to divide it into two parts: a part that generates potential moves, and a part that evaluates each move in terms of a set of criteria. The dichotomy between variation and selection was proposed by Darwin and Wallace as part of their theory of evolution by natural selection, but the distinction is more general: All adaptive, purposive behavior can be analyzed in this way. The dichotomy leads to two kinds of explanation for adaptive behavior: causal or mechanistic explanations, which define both the rules by which behaviors are generated (rules of variation) and the rules by which adaptive variants are selected (selection rules); and functional explanations, which just specify (perhaps in simplified form) the selection rules. Mechanistic accounts deal only in antecedent causes; functional accounts in terms of final outcomes. Thus the form of the shark is explained functionally by its hydrodynamic efficiency, the taking by a chess program of its opponent’s queen in terms of the improved position that results.
As we will see, the selection rules for learning cannot be stated as explicitly as the rule of natural selection. Indeed, even that rule is now much less clear than it was in days before we were aware of the problem of the unit of selection (individual organisms succeed or fail to reproduce, but it is individual genes that are passed on  what, then, is selected?). Consequently, functional explanations for adaptive behavior are often stated in terms of goals, purposes, or reinforcers (rewards and punishments), which act as guides of behavior. These notions can be for- malized in terms of some kind of optimality theory that makes goals explicit and shows how conflicting goals are to be reconciled. The general idea is that animals act so as to maximize something that relates to inclusive fitness, such as net rate of food acquisition, number of offspring, or territory size. I return to the relation between optimality accounts and selection rules in a moment.
Functional explanations can, in principle, be reduced to mechanistic ones: Given perfect understanding of the principles of genetics and development, and complete information about evolutionary history, we can, in principle, reconstruct the process by which the shark achieved its efficient form. For this reason the biologist Pittendrigh (1958) suggested the label teleonomic (as opposed to teleological) for such accounts. Teleological explanations are not acceptable because they imply final causation  the shark’s streamlining is teleologically explained by Mother Nature’s hydrodynamic foresight. Teleonomic accounts relate form and hydrodynamics through the mechanisms of variation and natural selection. Teleonomic functional accounts are philosophically respectable; teleological ones are not. In practice, of course, the necessary detailed information about mechanisms is often lacking so that we must settle for functional accounts and hope that they are teleonomic ones.
Functional explanations have sometimes been criticized as being “Just-so†stories, because they are so flexible  adaptive significance, or an unsuspected reward or punishment, can be conjured up to explain almost anything. There are two answers to this criticism: Functional explanations often lead to mechanistic explanations; and functional explanations can explain relationships that cannot be explained in any other way.
Functional accounts are often way stations to mechanistic explanations. In studies of learning they help identify important variables and draw attention to the constraints that limit animals' ability to attain functional goals. These constraints, in turn, provide clues to underlying mechanisms. For example, mammals and birds can easily learn to use stimuli as guides to the availability of food; a hungry pigeon has no difficulty learning that a peck on a red disk yields food whereas a peck on a blue disk does not. But they are much less capable of using past stimuli as guides. In the delayed-match-to-sample task, one of two stimuli is briefly presented, then after some delay both are presented, and a response to the one that matches the first is rewarded. Delays of more than a few seconds between sample and choice presentations impair gravely most animals’ ability to choose correctly. This is a memory constraint. Other psychological constraints have to do with animals’ ability to process information, and with their perceptual and motor abilities. Identification of limitations of this sort is the first step toward understanding behavioral mechanisms.
In addition to internal (psychological) constraints, there are also constraints imposed by the environment. For example, the animal cannot do more than one thing at a time, so that total amount of activity is limited; spatial arrangements limit the order in which food sites can be visited and the time between visits. Reinforcement schedules, either natural (as in picking up grain, one peck per grain, or in natural replenishment processes) or artificial (ratio and interval schedules, for example), further constrain the distribution of activities. Functional explanations, precisely expressed in the form of optimality theory, allow, indeed force, one to take account of these external constraints.
Functional explanations do one thing that no mechanistic explanation can: They can explain similar outcomes produced by different means. For example, the eyes of vertebrates and octopi are very similar in many ways: Both have lenses, a retina, and some means of limiting the amount of light that can enter. This convergence cannot be explained by a common ancestry or any similarity of developmental mechanisms. The only explanation we can offer for this astonishing similarity is the common function of these organs as optical image-formers. Because convergence is such a common phenomenon in evolutionary biology, it is no wonder that functional explanations are so common and so powerful there.
It is traditional in psychology to look down somewhat on functional accounts (although they often come in by the back door, in the form of vaguely expressed reinforcement theories). Indeed, one of our most influential figures boasts in his memoirs that in planning his major work he deliberately avoided any discussion of adaptiveness. Few maintain that position today. Looking at behavior in terms both of its adaptive (evolutionary) function and in relation to current goals (reinforcers) is useful in identifying important variables and in distinguishing environmental from psychological constraints. Functional and mechanistic theories are on an equal footing in this book.
The idea that organisms attain goals, either through natural selection for the best form of wing or individual reinforcement of the most effective foraging strategy, derives naturally from the selection/variation idea: A wide range of variants occurs, the best (in terms of flight efficiency or eating frequency) are preferentially selected, the next round of variants contains a few that do even better, and so on. This process will, indeed, lead to better adaptation only if two things are true: We have the selection rule right – that better fliers really have more offspring; and that the right variants occur. In other words, an animal may fail to behave in what seems to us the optimal fashion either if we have misread what it is trying to achieve (the selection rule), or because it never generates the necessary behavioral variant: The most efficient foraging strategy cannot be selected (reinforced) if it never occurs. Memory constrains behavior in ways that prevent animals from developing certain kinds of foraging patterns – patterns that require memorization of complicated sequences, for example. These patterns will not occur, even in situations where they would be optimal. Thus, failures to optimize are, if anything, even more informative than successes, because they offer clues to the underlying behavioral mechanisms. Optimality theories to explain how animals adapt to reward and punishment are discussed in Chapters 6-10.
EVOLUTION AND DEVELOPMENT
The processes of individual development, ontogeny, are the product of past evolution and they also limit future evolutionary possibilities. Unlike human machines, natural machines – animals and plants – manufacture themselves. This process limits their potential and often incorporates the effects of experience in ways that contrast with, and thus help define, learning.
Organisms change throughout their lifetimes, and the processes by which they change are the outcome of past evolution. As Darwin pointed out, organisms bear their evolutionary history both in their structure and in the manner of its development. Rudimentary organs provide some of the most striking examples. The human vermiform appendix, the rudimentary breasts of male mammals, the vestigial lung of snakes (that have only one functional lung), the teeth of fetal whales that vanish in the adult, the uncut teeth of unborn calves  none has any function in the adult, yet they remain: “They may be compared with the letters in a word, still retained in the spelling, but become useless in the pronunciation, but which serve as a clue for its derivation.â€Â5 There are behavioral parallels in the inappropriate “grass-flattening†of domestic dogs, and exaggerated fears (of the dark, or of strangers, for example) in human children. In many cases these vestigial behaviors disappear with age, as in some of Darwin’s examples.
These examples illustrate the half-truth that ontogeny recapitulates phylogeny, that is, the idea that the stages through which an organism passes, from embryo to adult, represent a history of the race in an abbreviated form. Gill slits in the human fetus were once taken to mean that the fetus at that stage resembles the ancient fish from which mammals are descended. The actual relations between ontogeny and phylogeny are more complicated and derive from the fact that evolution acts via the mechanisms of development.
Development can be compared to a railroad switchyard in which incoming cars on a single track are sorted by a branching arrangement of switchoffs so that each car arrives at a different destination. At conception the organism is essentially undifferentiated and “pluripotent,†that is, many things are possible (these are the “stem cells†that have played a role in recent political controversy about what is permissible research). With progressive cell divisions, there is increasing differentiation and the options for further development are reduced: the railroad car has passed through several switchoffs and is closer to its final destination. This process of progressively finer differentiation, and the concomitant reduction in future options, takes place throughout life. Eventually the car enters the final stretch of track that terminates in death – which is not a wearing out, but the largely predetermined end of a course charted by prior evolution.
Typical life span, like other characteristics, is determined by its costs and benefits, weighed in the delicate balance of natural selection.
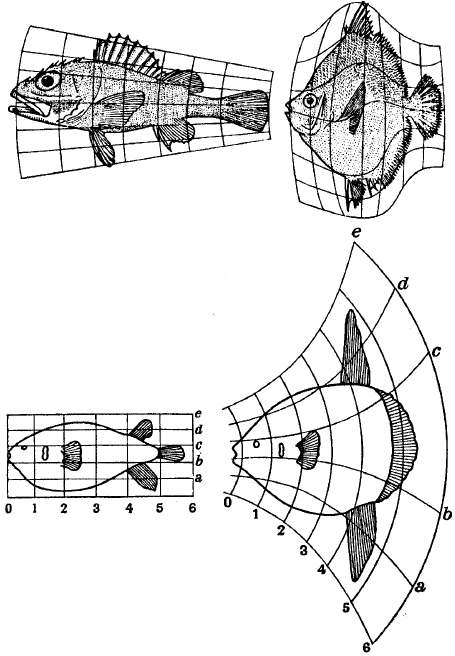
Genes determine the direction of the successive switches that occur throughout ontogeny. We don’t yet know exactly how this works: “Despite our relatively detailed understanding of molecular biology, the processes which control the development of a multicellular organism from a single cell, the fertilized egg, are almost completely unknown†(Caplan & Ordahl, p. 120, wrote this in 1978, but our ignorance is still profound). Nevertheless, one thing is clear: The genetic changes that provide the raw material for evolution act not directly on morphology or behavior, but on the course of development – a stage may be added or missed entirely, stages may be accelerated or retarded. These changes in the path of development are the raw material for the formation of new species. For example, if the genital system matures relatively faster than the rest of the body, the result may be a sexually mature “larval†animal, as in the case of the Mexican axolotl (Ambystoma tigrinum), a salamander that can become sexually mature while still a tadpole. Continued selection might well fix a change of this sort, so that the terrestrial stage is completely abolished and a new species of entirely aquatic amphibian is the result.
It is easy to see that this process will leave traces of a species’ past evolutionary history in the path of development of an individual organism. For example, the immature form of a fish such as the angelfish (Pterophyllum scalare), which is strongly laterally compressed in the adult, or of flatfish such as the flounder (Bothus lunatus), which is vertically compressed and has lost bilateral symmetry by having both eyes on the same side of the head, is quite normal looking, with the “typical†elongated, bilaterally symmetrical fish shape. Presumably the abnormal body form arose via genetic changes that acted to modify growth gradients in the ancestral species at a relatively late stage of development. Thus the immature forms of these “abnormal†species provide a partial record of the immature forms of the ancestral species from which they derive.
This view of evolutionary action implies that related species with different bodily forms should often be transformable one into the other by stretching or compressing along the three bodily axes. This was first pointed out by the British biologist D’Arcy Wentworth Thompson; Figure 1.1 shows a couple of fish examples.
The action of a gene depends on its environment, which includes the rest of the genotype, the cell of which it is a part, the constitution of neighboring cells, circulating metabolites such as hormones, and the neurotransmitters released by nerve impulses. For example, during the development of the fruit fly Drosophila a specific section of cytoplasm (the polar cytoplasm) influences the nuclei that migrate through it to differentiate into the reproductive cells. If the polar cytoplasm is removed from the egg so that migrating nuclei do not encounter it, the reproductive cells do not develop and a sterile animal is the result. Polar cytoplasm affects the expression during development of genes responsible for the reproductive system.
Because the organism’s internal environment is affected intimately by its external environment, the course of development is a joint product of genotype and the environment in which the organism grows up. In effect, therefore, the action of genes and the action of the environment are symmetrical: Each depends on the other. The successive switches that constitute development are joint effects of environment and genotype. The main difference between environmental and genetic effects is that, since the genotype is fixed but the environment can vary, environmental effects on behavior may be reversible. Because the sensorimotor systems that bring the animal into contact with its environment develop with age, the effects of environment on development are likely to become richer and more subtle as the organism grows older. The effects of the environment on an embryo or a newborn may be great, but they are unlikely to involve the transmission of as much information as interactions later in life. On the other hand, environmental effects are likely to be self-limiting owing to the accumulation of irreversible changes, so that mid-life may often be the time of maximal sensitivity to external influences.
Gross morphological changes are not readily reversible. This is why traces of an organism’s evolution are retained in its development. But behavior, and presumably the changes in brain state that correspond to behavior, is, almost by definition, easily altered. Consequently it is not at all clear what we should expect of the relation between behavioral and morphological development. Is it reasonable to assume, for example, that behaviors that appear early in ontogeny only to disappear later tell us the same sort of thing as human fetal gill-slits? Were these the behaviors of our immature ape ancestors? In the case of some primitive reflexes this may be a reasonable guess. For things like fear of strangers or of the dark, we cannot be sure6. This question will not be settled until we understand how brain structure and physiology relate to behavior. How is past experience represented neurophysiologically? How does the current environment interact with this representation, and the animal’s motivational state, to produce action? Unfortunately, we are a very long way from answering these questions.
EPIGENESIS AND GENETIC ASSIMILATION
The subtleties of gene environment interaction are illustrated nicely by some ingenious experiments by the geneticist Waddington. Using fruit flies (Drosophila melanogaster), Waddington showed that environmental changes can act as a sort of probe to uncover latent characteristics of the genotype. In one experiment, fruit-fly pupae were subjected to heat shock at an age when this treatment was known to produce adults with various kinds of altered wing-vein pattern (these variants are called venation phenocopies, see Figure 1.2). Individuals showing a particular kind of phenocopy were then selectively bred together. Soon, strains were produced that responded to this kind of stress with high frequencies of the phenocopy. Continued intense selection made it possible to produce strains in which the selected-for abnormality appeared even in the absence of heat stress. Thus, phenotypic variants produced by an environmental probe, when bred together, eventually yield genotypes that show the variants even without any probe. Waddington called this effect genetic assimilation.
There are many other examples. Consider the familiar phenomenon of the formation of skin calluses in response to (and as a protection against) abrasion. Calluses form much more readily on some parts of the body, such as the hands and feet, than on others – reflecting differential selection pressure. Indeed, calluses will form on the feet of a bedridden person (i.e., in the absence of the usual environmental stimulus). The phenomenon even shows an evolutionary vestige, in the form of knuckle calluses, which form spontaneously in many individuals. They are now of no use, but presumably served an adaptive function in our knuckle-walking ancestors. Evidently past selection for callus development in response to abrasion leads, in some people, to the spontaneous development of calluses7.
In higher animals, training procedures may be regarded as genetic probes whose effects can be interpreted in the same way as Waddington’s heat shock. For example, the ease with which an individual learns some skill, such as music or mathematics, may be an indication of how close his genotype is to one that would produce this behavior with minimal or no training. One might speculate that a program of selective breeding for precocious musical ability would eventually lead to Mozarts capable of writing sonatas at six, and finally, perhaps, to infants capable of spontaneous musical expression of a high order in the absence of any explicit training8.
This may seem improbable, either because it seems unreasonable that something as complex as musical proficiency should be entirely innate, or because we are accustomed to think of learned and innate as opposites. There is no basis for either objection. For example, sheep dogs are selected for their ability to learn to herd sheep; and training a professional sheep dog takes years. Nevertheless, components of herding, such as circling and attempting to group people or animals, appear spontaneously in pets that have never been specifically trained. Speech is learned, yet adult intonation patterns, and an enormous number of phonemes (speech-sound units), occur spontaneously in infants. Precocial birds learn to identify their own species early in life, via the process termed imprinting; nevertheless, the type of object accepted for imprinting, especially later sexual imprinting, cannot deviate too much from the natural stimulus if it is to be effective. Young male swamp sparrows (Melospiza georgiana) learn their song from adult males, but are quite selective about what they will accept as a model. In all these cases, the learned ability is put together with ingredients provided innately.
Very complex behavior may be innately programmed, and complexity by itself is an unreliable guide as to whether something is learned or innate. Almost all the intricate behavior of insects develops independently of experience, for example. Some songbirds, such as the song sparrow (Melospiza melodia), develop their elaborate song with almost no specific experience, whereas others, such as the chaffinch (Fringilla coelebs), require early exposure to their much simpler species’ song if they are later to sing normally.
Most dramatic of all, perhaps, is the inheritance of navigational ability by migratory birds. The golden plover (Pluvialis dominicus) breeds in northern Alaska and migrates during the fall to Argentina by way of Labrador, then returns in the spring across land, over Central America heading north and west along the Mississippi River. The bobolink (Dolichnyx oryzivorus) travels from Maine to Brazil; Wilson’s petrel (Oceanites oceanicus) from the Falkland Islands (near the Antarctic) to Newfoundland. Some marine animals, for example, whales and salmon, perform comparable feats of migration. Migratory birds use such navigational-aid cues as sun direction, assessed both directly and through sky polarization on partly cloudy days; time (via an internal clock keyed to the light-dark cycle); the direction of the earth’s magnetic field; very low-frequency sounds (infrasound), such as the sound of surf on a distant beach (which may be audible hundreds of miles away); visual-terrain cues; and perhaps other features not yet identified. These cues are combined to guide flight in ways that are far from being understood, even in the short flights of homing pigeons. The capacity to carry out these long migrations is but little dependent on experience in many solitary species. The complexity of a behavior pattern is tells us little about its developmental origins.
Environmental effects on development can occur at any time, from conception to old age. For example, experiments by the pioneering developmental psychobiologist Zing-Yang Kuo showed that the passive movement of the head and beak in embryonic chicks caused by their heartbeat in the egg plays a role in the later development of pecking. Gottlieb has demonstrated that ducklings’ ability to follow the repetitive call of their mother depends for its selectivity on pre-hatching experience with sound frequencies above 1500 Hz. Normal ducklings, given a choice between a recording of the normal call and the call with the higher frequencies filtered out, reliably chose the normal call. However, birds deprived of hearing the mother while in the egg choose both alternatives equally. Under normal circumstances all ducklings receive the required experience, both by hearing their own calls while in the egg, and by hearing the calls of their siblings in adjacent eggs9.
These dependencies of later behavior on apparently unrelated earlier experience may seem odd and even capricious, but they fit in well with the epigenetic view of development: the now well-accepted idea that development is the outcome of continuous interactions between a genetically encoded program and the environment of the developing organism, rather than the unfolding of a preformed and predetermined entity. Gene action is a strictly conditional business, dependent on the gene environment and thus, in many cases, on the environment of the organism. Natural selection favors any genetic change that reliably has a beneficial effect on the phenotype. If the expression of that genetic change in the phenotype depends on the presence of a particular environmental feature, then as long as that feature is a reliable accompaniment of normal development, the gene will be favored and an environmental dependence will become established.
Imprinting is the best-known example of this kind of dependency. Precocial birds, such as chicks and ducklings, will generally form a permanent attachment to individuals that they see and can follow during the first day or two of life. This is part of the process by which these species learn to identify their own kind. Species identification in these animals might have developed in several ways. For example, the ducklings might be provided at hatching with an essentially built-in “template†enabling them immediately to recognize conspecifics. Many species are provided with such a template – almost all insects, and brood parasites such as the cuckoo (Cuculus canorus) and North American cowbird (Molothrus ater), which never see their own parents and could not function without the innate ability to recognize their own species. But even with such a template, the ducklings would also require a propensity to follow (following the mother when she calls is essential if the duckling is not to end up inside a predator). Following the mother implies some learning so that the animal doesn’t follow any female duck. But given the existence of some learning mechanism, it is obviously parsimonious to arrange that the young animals learn not only the particular individual that they must follow, but also the characteristics of that individual’s species. Under normal circumstances, of course, the first individual that the chick or duckling sees is a member of its own species. If not, its future is likely to be dim; only individuals whose first experience is of their own parents are likely to contribute to future generations. An efficient solution to the species identification problem in precocial species, therefore, is the existence of a critical period during the first days of life when the individual learns about the characteristics of its parents by following them. Most genes act only during specific stages of development, so that small changes in genotype may have been necessary to change from the built-in template kind of development to imprinting. As imprinting evolved, less and less of the template would be necessary and mutations tending to degrade it would not be selected against10.
Vestiges of the template mechanisms from which imprinting may have evolved can still be detected. Ducklings imprint most rapidly to stimuli resembling members of their own species, and weak imprinting to a severely abnormal stimulus, such as a moving box, can be overcome later by exposure to the natural stimulus. I return to imprinting in Chapter 13.
SUMMARY
The introductory chapter of any book must guess at what readers already know. I have assumed that many readers will know somewhat more about psychology than biology, but will still hold (one hopes weakly) commonsense mentalist views about the causes of human and animal behav- ior. Hence my emphasis on animals as machines, and the discussion of intentional terms and their uncertain status as explanations of behavior. Some other readers are likely to have encountered the extreme ethological dogma that insists on the uniqueness of each species and the consequent impossibility of a general psychology of learning. Hence, it seemed important to note the similarities among niches and the constraints that limit all information processing systems, because these provide the basis for such a general psychology.
The distinction between learned versus innate behavior is another commonplace that requires modification. Some years ago, a perceptive psychologist (Verplanck, 1955) wrote a paper entitled “Since learned behavior is innate, and vice versa, what now?†that defined the problem: Learning depends upon inherited mechanisms and is constrained by them. Moreover, past experience affects later behavior in many ways, only a few of which we call “learning.†The best way to get a feeling for the range of possibilities is to look at ontogeny, how it reflects past evolution, and how it incorporates the effects of environment, sometimes in an apparently capricious and idiosyncratic way. All this leads to the epigenetic view of development: Changes in morphology and behavior during ontogeny reflect a process of differentiation in which some options are chosen and others given up, guided at every instant by the joint effects of genotype and environment.
Beyond that, the chapter sets the stage for those that follow by distinguishing functional from mechanistic explanations and showing why each is useful. Optimality theory allows functional explanations to be formulated precisely and forces us to specify the things that are important to an animal, as well as the internal and external constraints that limit the range of behavioral variation. These constraints, in turn, provide clues to the underlying mechanisms that allow the animal to behave in a goal-directed way.
The next chapter begins the story of how animals can adapt to a variable world by describing the very simplest adaptive mechanisms: the processes that plants and single-celled animals use to find a congenial habitat, and the automatic, protective reflexes of higher animals.
NOTES
Note 1
Niche is one of those essentially undefinable terms that are nevertheless essential to what might be termed the “sciences of organized complexity,†such as ecology, psychology, and behavioral biology. Like most such terms, it is best defined by example. It is pretty obvious that the talents required of a good leopard are quite different from those needed by an effective antelope. Among the former are powerful means of attack, a digestive system attuned to meat, and a visual system adapted to attend to one thing at a time. Among the latter are a good means of evading attack, a lengthy gut able to cope with the poor diet provided by grazing, and a visual system able to detect threat from any quarter. Thus, the claws and teeth of the leopard, its forward-facing eyes and short digestive tract, as well as the rapid and maneuverable running of the antelope, its lengthy digestive tract and sideways-facing eyes, all have an obvious functional explanation.
The behavioral adaptations required by different niches are usually less apparent than morphological differences, especially if they involve differences in the way that past experience affects present potential. The match between adaptation and niche is no less close because it is hard to see, however.
The basis for the modern idea of niche is Darwin’s discussion of an organism’s “place in the economy of nature.†References to later work, including mathematical definitions of the concept, can be found in any standard ecology text.
Note 2
The division of interest between those features of adaptive behavior that differ among niches and those that are common to all niches corresponds roughly to the division between ethologists and animal psychologists. In days when psychologists were less aware of biology than they are now, learning theorists rallied around the search for “general laws of learning." The discovery of types of learning specific to particular situations or species gradually made this position untenable. There are general laws, but they seem to reflect commonalities among niches or general features of all information-processing systems, rather than a common plan of construction – as the earlier view implied. In biological terms, the resemblances are a mixture of convergence and homology (the composition of the mixture being largely unknown in most cases) rather than the pure homology implied by the general-law idea (see also note 6).
Note 3
Excellent, readable accounts of intentional systems, chess-playing machines, and the like, appear in the philosopher Daniel Dennett’ s book Brainstorms (1978), a collection of essays on psychology and artificial intelligence.
Note 4
Of course, human psychology must eventually come up with an explanation for why intentional terms are so useful and ubiquitous as makeshift explanations. Perhaps the answer is that intentional accounts are just fuzzy functional explanations, and thus the best that one can do without detailed knowledge of behavioral mechanisms.
Methodological behaviorism is usually contrasted with radical behaviorism, the position advocated most forcefully by B. F. Skinner. Radical behaviorism asserts that it is unnecessary to go significantly beyond the level of behavioral description to account for all behavior. The position made some sense in reaction against rampant mentalism, but makes none now. To pursue the computer analogy, it is like asserting that the chess-playing program can be explained en- tirely in terms of its inputs and outputs and direct (stimulus-response) links between them. For critiques (sympathetic and otherwise) of various aspects of behaviorism see Chomsky (1959), Dennett (1978), and Staddon (1967, 1973; 2001a).
The philosophy of behaviorism was crystallized by a book by John Broadus Watson, Psychology from the Standpoint of a Behaviorist (1919), a polemical attack on the then prevailing phenomenological view that took subjective experience (with all its problems of intersubjective reliability) more or less at face value. This book is the source of a dominant movement in recent American psychology. It has given rise to a number of subsidiary streams, from Skinner’s radical behaviorism, at one extreme, to various eclectic movements that are willing to explain human and animal behavior in terms of expectancies, attitudes, “means-end-readinesses†and the like. Good accounts of these historical trends are available in a number of books, most notably Boring (1957) and Herrnstein and Boring (1965). For a witty, clear, and controversial account of the antecedents of these movements see Bertrand Russell’s marvelous History of Western Phi- losophy (1946).
Note 5
Darwin (1872) p. 525. The German biologist Ernst Haeckel (1834-1919) was one of the first (Darwin preceded him) to make much of the relations between development and evolution, al- though his views are in many ways too simple and lent themselves to the “ontogeny recapitulates phylogeny†parody. Gould (1977) has summarized the modern view as follows: “Evolution oc- curs when ontogeny is altered in one of two ways: when new characters are introduced at any stage of development with varying effects upon subsequent stages, or when characters already present undergo changes in developmental timing†(p. 4). The classic work on the development of morphology is D’Arcy Wentworth Thompson’s On Growth and Form (1917), a wide-ranging book with a strong esthetic element, written at a time when original scientific writing was meant for the general reader. A definitive account of quantitative issues is Julian Huxley’s Problems of Relative Growth (1932). Important approaches to the development of behavior are due to Kuo (1967, 1970) and Lehrman (1970).
For an excellent account of current views on the mechanisms of evolution and development see the description of a Dahlem conference on the subject by Lewin (1981) in Science magazine.
Note 6
The problem here is an instance of the well-known difficulty of deciding whether characters are homologous (reflect common ancestry) or analogous (reflect convergent selection pressures). The human hand and the bat’s wing are homologous structures, but the eyes of mammals and octopi reflect convergent selection pressures  although recent genetic discoveries are raising questions about this. A behavior such as suckling is obviously adaptive only at a certain time during a mammal’s life; hence its early appearance and subsequent disappearance need not reflect similar behavior in non-mammalian ancestors. As Darwin pointed out, the more useless and apparently irrelevant the character, the more useful it is as a guide to evolutionary origins.
Note 7
Genetic assimilation at first achieved notoriety because of its obvious resemblance to Lamarckian inheritance of acquired characteristics: The venation phenocopies are environmentally induced, yet selection of individual animals showing them eventually leads to spontaneous appearance of the effect. In fact, no direct effect of environment on genotype need be assumed. But the effect makes Darwinian sense if we assume that the subset of animals showing the venation effect of heat stress has genotypes genetically “closer†to the genotype necessary for its spontaneous appearance than the rest. This assumption, plus the assumption of essentially random genetic variation about the mean, is sufficient to account for the effect of selection in shifting the mean genotype to the point where venation effects appear spontaneously. Waddington’s “epigenetic landscape†and his genetic assimilation experiments are described in a 1956 paper and in a number of general accounts (e.g., 1962).
Note 8
The allusion to Mozart is originally due to Darwin, who pointed out that “if Mozart, instead of playing the pianoforte at three years old with wonderfully little practice, had played a tune with no practice at all, he might truly be said to have done so instinctively†(1872, p. 267). The phenomenon, however, is not uncommon. Consider the great mathematician Blaise Pascal (1623-1662), who because he was frail as a child was protected by his parents from the excessive intellectual excitement evoked in him by Euclid. Shielded from Euclid, young Pascal reinvented much of geometry on his own. His sister Gilberte wrote “. . . Since my father has been so careful to conceal all these things [mathematics] from him that he [Blaise] was forced to invent his own names. Thus, he called a circle a ‘round,’ a line a rod and similarly for all the rest. Using these names he set up axioms and finally complete proofs. And since, in these matters, one proceeds from one thing to another, he continued to make progress and pushed his investigations to the point where he reached the 32nd proposition of Book I of Euclid…†(Meschowski, 1964, p. 34). Pascal obviously did not need a great deal of specific experience to learn the primitives of geometry.
The key issue in the nature-nurture controversy is where the relevant information comes from. There are only two alternatives: If an organism shows some organized structure or behavior, the information must have been communicated either during phylogeny (when we are inclined to term the behavior instinctive) or during ontogeny (when it is termed learned) or, more probably, both. By any reasonable criterion it seems that Pascal’s knowledge of geometry was largely instinctive. See Staddon (1981a) for a speculative extension of these ideas to the relation between cultural and genetical evolution.
Note 9
Calls made in the egg serve the vital function of synchronizing hatching – which is beneficial to all because it simplifies the logistics of feeding versus incubation by the parents. Gottlieb’s work is described in his book (1971) and papers (e.g., 1974).
Note 10
The term template has been most extensively used by Peter Marler and his associates in their elegant studies of the ontogeny of song learning in the white-crowned sparrow (Zonotrichia leu- cophrys; see e.g., Marler & Hamilton, 1966). They used it to refer to the “model†set up in the male by early experience of the adult song to which its own singing will later be matched. Strictly speaking, a template is a simple but literal model of some stimulus feature that can be acquired by experience, as in the sparrows, or is more or less built in.
The term template is an attempt to label the process of encoding by which any organism must abstract information about the external world. No representation, even a color photograph or movie, is complete; moreover, the more literal and complete a representation, the more memory storage it requires. Hence, natural selection has strongly favored the elimination of redundancy in the formation of templates: Features that are not reliable predictors of the object to be identified are eliminated; reliable features, especially if they are simple, are retained. Thus, the male English robin (Erithacus rubecula) recognizes other males simply as red fluffy objects of a certain size; for the male stickleback (Gasterosteus aculeatus) another male is any red object subtending a certain visual angle.
Information on imprinting is widely available. Good secondary sources are Hinde (1970), Bateson (1974) and Shettleworth (1998). The original work was done by Heinroth (1911) and his student Konrad Lorenz (1935, reprinted and translated in Lorenz, 1970). Related effects of early experience are discussed in Chapter 14.
Edit Notes
- 2005-08-11 Transcribed text by copy-and-paste from PDF version; one illustration retrieved by copy-and-paste, one illustration yet to be copied. Are illustrations copyrighted?